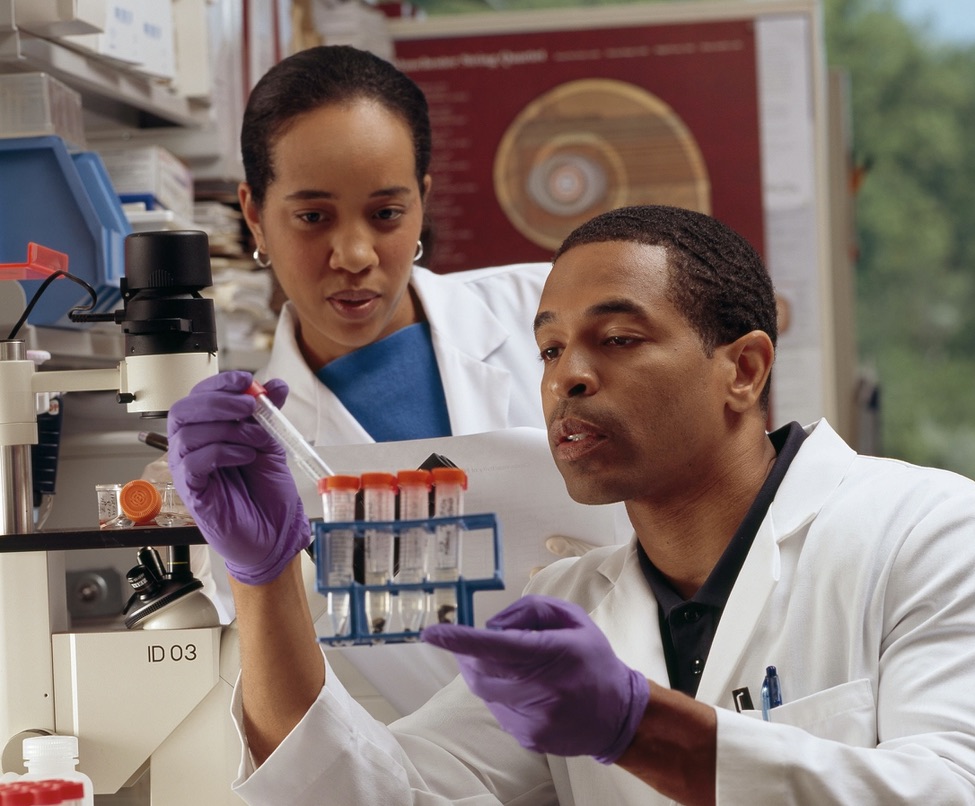
Gene therapies offer massive therapeutic potential and the possibility of making clinicians' dreams of treating diseases a reality: the ability to cure genetic conditions with a one-time treatment.
Gene therapies introduce genetic material into a patient’s cells to correct or compensate for a genetic defect. For instance, hereditary blindness can be caused by biallelic mutations in the gene RPE65, which reduce or eliminate RPE65 activity, leading to loss of vision in childhood or adolescence. The first FDA-approved gene therapy, Luxturna (voretigene neparvovec-rzyl), delivers a normal version of the RPE65 gene directly into retinal cells, producing wild-type levels of RPE65 functioning and restoring vision loss.1
This success in 2017 opened the floodgates to gene therapy development. There is great potential to treat various inherited or acquired genetic disorders, including cancer. The activity over the past few years has led to the recent landmark approval of the first CRISPR-based gene therapy for sickle cell disease and transfusion-dependent beta-thalassemia.2
While extremely promising, one of the most challenging aspects of developing a gene therapy is designing and producing a viral vector that contains the complete transgene for introduction into a cell’s nucleus. Below, we give an overview of which viral vectors are used in gene therapy development, the advantages and drawbacks of each, and how artificial intelligence is used to overcome obstacles in viral vector production.
What are Viral Vectors?
A viral vector is a virus genetically modified to deliver a specific transgene or piece of genetic material into a host cell. They are commonly used in gene therapy and vaccine development, as they can effectively transfer genetic material into cells in a controlled manner. Several types of viral vectors can be chosen depending on desired expression level, size of the transgene, the cell type that needs to be targeted, and other delivery parameters.
What is a vector in gene therapy?
In gene therapy, a vector is a delivery vehicle used to transport therapeutic genetic material into target cells. Vectors can be derived from viruses or non-viral systems engineered to deliver genetic payloads efficiently. These vectors are designed to ensure therapeutic genes' safe and effective transfer into the patient's cells. The choice of vector plays a crucial role in determining the success and safety of gene therapy interventions.
Common Types of Viruses Employed as Vectors to Deliver Gene Therapies
Viral vectors successfully used in the preclinical and clinical development of gene therapies include adenoviruses, adeno-associated viruses (AAVs), lentiviruses, and retroviruses.3
Adenovirus (ADV) vectors
ADV is a non-enveloped virus that commonly causes upper respiratory tract infections.3 There are hundreds of human ADV genotypes, and the infection cycle has been heavily studied and is known to transiently transduce nearly any mammalian cell type. The virus rarely causes severe infection in most healthy children and adults. Those infected carry lifelong immunity and express ADV-specific neutralizing antibodies.3
Adeno-associated viruses (AAV) vectors
AAVs are small, non-enveloped viruses that carry single-stranded DNA. It doesn’t cause any known human disease, yet it has been a popular laboratory tool as it has a relatively simple genome and can be easily manipulated. Thousands of AAV variants have been classified into a few serotypes, with differential affinities to a range of cell surface receptors.3
Lentivirus (LV) vectors
LVs are complex retroviruses that are spherical, enveloped, and contain a single-stranded RNA genome. The viral particle fuses with the cell membrane upon binding to cell surface receptors, and the genome is released into the cytoplasm. Following reverse transcription, the genome is integrated into the host genome in a non-random way, favoring transcriptionally-active sites.3
The Use of Viral Vectors in Gene Therapy Clinical Trials
The viral vectors mentioned above have been studied extensively in preclinical and clinical studies, with varying degrees of success. Currently, ADVs, AAVs, and LVs are all being investigated clinically for certain therapeutic indications.
Adenoviral vectors in clinical trials
Adenoviral vectors are widely used in current gene therapy clinical trials and have the longest history in gene therapy, dating back to the infamous case of Jesse Gelsinger.4 This tragedy slowed the development of gene therapies for years and raised awareness about the severe innate immune response that can be generated by ADVs (specifically the capsid protein).
Nevertheless, for therapies where an immunological response is beneficial, ADV vectors showed promise: In 2003, Gendicine (an ADV vector expressing the p53 gene) was approved in China, becoming the first-ever gene therapy for cancer.3
Currently, they are being investigated clinically for novel vaccine development against infectious diseases and as cancer therapies. ADV-based vaccines against Ebola, influenza, HIV-1, and SARS-CoV-2 are all in ongoing clinical trials but haven’t navigated regulatory approval.3 ADV-based anti-cancer strategies, including delivery of suicide or regulatory genes, are also under clinical investigation.
Recently, the FDA approved the first gene therapy based on an adenoviral vector: In December 2022, Adstiladrin was approved for the treatment of high-risk non-muscle invasive bladder cancer resistant to Bacillus Calmette-Guérin.5
AAV vectors in clinical trials
AAV-based gene therapies are being investigated in several rare diseases and there have been over 200 clinical trials worldwide using AAVs.2 Due to small patient populations and limited enrollment, clinical study of AAV-based therapies is slow, limited to diseases with no other treatment options. Clinical trials are ongoing for age-related and diabetic macular degeneration (AMD), muscular dystrophies, hemophilia, and X-Linked myotubular myopathy (XLMTM).
While some trials have produced promising results, there have also been several noteworthy trials that have been halted due to safety concerns: A high-dose AAV vector for treating XLMTM was recently linked to the deaths of three patients.6 These challenges will need to be addressed should AAV therapies continue to be commercialized.
The FDA has approved three AAV-based therapies (as of January 2023): Luxturna for retinal dystrophy, Zolgensma for spinal muscular atrophy, and recently, Hemgenix for the treatment of adults with Hemophilia B (congenital Factor IX deficiency).1,7,8
Lentiviral vectors in clinical trials
LV vectors have been primarily used for engineering ex vivo gene therapies, such as generating chimeric antigen receptor T (CAR-T) cells.3 The first FDA-approved ex vivo gene therapy, Kymriah, transduced autologous T cells with an LV vector carrying a CAR transgene that binds CD19 and was used to treat pediatric B-cell relapsed and refractory acute lymphoblastic leukemia.9 Other ex vivo therapies that target different cancers are being investigated, and as proof of the safety of LV vectors becomes available, the number of trials will continue to increase.3
The Advantages and Challenges of the Different Viral Vectors for Gene Therapy
Using ADV, AAV, and LV vectors in gene therapy development has distinct advantages and drawbacks. Choosing the right vector type depends on the transgene, desired expression level, target cell type, and therapeutic application.3
Adenoviral vectors in Gene Therapy
ADV vectors are often the preferred choice for gene therapies due to their high transduction efficiency, stability in host cells, capacity (up to 4 kb for first generation, up to 10kb for second generation and up to 36 kb for third-generation vectors), and ability to target various tissues2.
However, ADVs also have some significant drawbacks. Many people have been previously infected with ADVs and therefore have pre-existing immunity that can lead to strong immune responses to the viral vector or transgene products.3 These safety concerns limit the use of ADVs to diseases that are not impacted by immune responses or even rely on the target cells being killed (e.g., in cancer treatment).
AAV vectors in Gene Therapy
AAVs are versatile and stable, with a lifespan of over 10 years in differentiated cell populations.10 However, AAVs have a limited capacity of around 5 kb, which has led to developing strategies such as split vector approaches to address this limitation.
Like ADVs, AAVs can also cause immune responses that must be considered during discovery and development. The immunogenicity of AAV-based gene therapies can increase due to production impurities, which include empty capsids and encapsidated, non-therapeutic DNA.11 These issues have led to stalled clinical development of several AAV-based gene therapies. Additionally, converting the single-stranded DNA genome into double-strand DNA, which is required for transduction in host cells, can be a rate-limiting step.
AAVs can also be difficult for tissue-specific transgene expression, and their production can be challenging to scale up economically.
Lentivirus vectors in Gene Therapy
LVs are often used to deliver transgenes due to their ability to integrate into the host genome effectively, express multiple transgenes (up to 9 kb) for long periods from a single vector, and infect non-dividing cells.12 They also tend to have a low immunogenicity profile.
However, there is a risk of generating a replication-competent virus, particularly with LVs derived from HIV-1. To address this risk, third-generation vectors have been developed that do not have the genes required for genome packaging. Still, LVs have high mutation and recombination rates, which can pose a small but significant risk. One primary concern is the accidental generation of an oncogene, which is why LV vectors are only used in ex vivo applications, such as CAR-T therapy, and not in vivo therapies.
Conclusion
Viral vectors for gene therapy play a crucial role in developing these therapeutics. Different types of viral vectors can be used depending on the specific requirements of the therapy. ADVs, LVs, and AAVs have all been used successfully in preclinical and clinical gene therapy trials.
The viral vector design and manufacturing challenges currently in the way of accelerated gene therapy development are now being tackled using artificial intelligence. Form Bio is empowering AAV gene therapy developers with FORMsightAI, a custom, in silico program to accelerate drug development and manufacturing. Our computational solutions help overcome development barriers by efficiently exploring AAV vector design space and assessing the quality of AAV bioreactor runs.
Interested to learn how Form Bio’s artificial intelligence strategies will accelerate your gene development program?
Schedule Your Discovery Call TodayReferences
- DA approves novel gene therapy to treat patients with a rare form of inherited vision loss. FDA website. Accessed January 10, 2023. Published December 18, 2017
- The First CRISPR Drug: Vertex Pharmaceuticals’ Casgevy Wins U.K. Approval for Sickle Cell Disease. Genetic Engineering and Biotechnology News website. Accessed December 4, 2023. Published November 16, 2023
- Bulcha J.T. Viral vector platforms within the gene therapy landscape. Sig Transduct Target Ther. 6, 53 (2021)
- Raper SE, Chirmule N, Lee FS, et al. Fatal systemic inflammatory response syndrome in a ornithine transcarbamylase deficient patient following adenoviral gene transfer. Mol Genet Metab. 80(1-2):148-158 (2003)
- FDA approves first adenoviral vector-based gene therapy for high-risk Bacillus Calmette-Guérin unresponsive non-muscle invasive bladder cancer. FDA website. Accessed January 11, 2023.Published December 19, 2022.
- Gene Therapy: It’s Time to Talk about High-Dose AAV. GEN website. Accessed January 10, 2023. Published July 7, 2020.
- FDA approves innovative gene therapy to treat pediatric patients with spinal muscular atrophy, a rare disease and leading genetic cause of infant mortality. FDA website. Accessed January 10, 2023. Published May 24, 2019.
- FDA Approves First Gene Therapy to Treat Adults with Hemophilia B. FDA website. Accessed January 10, 2023. Published November 22, 2022.
- FDA approval brings first gene therapy to the United States. FDA website. Accessed February 11, 2023. Published August 30, 2017
- Au H.K.E. Gene Therapy Advances: A Meta-Analysis of AAV Usage in Clinical Settings. Front. Med. (2022)
- Distinguishing AAV Empty and Fragmented Capsids: Closing the Gene Therapy Accessibility Gap with Improved Manufacturability. Form Bio website. Accessed December 4, 2023. Published August 23, 2023
- Sanyal S. The Pros and Cons of Lentiviral and Adeno-Associated Viral Vectors. The MedicineMaker. Published October 7, 2022. Accessed January 7, 2023.
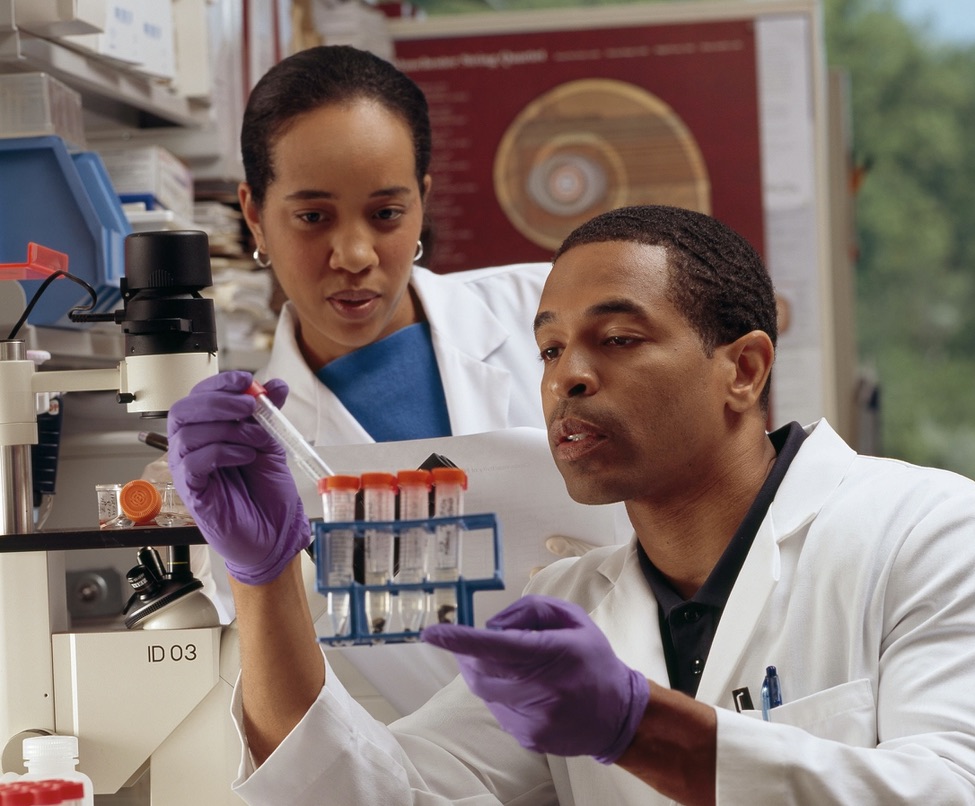